The energy transition will be accompanied by a materials transition
Abundance, concentration, and cost are the keys to the materials transition
Questions
What other industries will be affected by the energy transition?
What are the common sources of innovation in the materials transition?
Why do the economics of the startups in this space make sense now?
Background
I have been working to better understand the technological and business landscape associated with the energy transition. In the energy transition, we are not only changing the forms of energy that we use (renewable electricity instead of fossil fuels), but also we are changing the materials associated with those forms of energy. Overcoming the intermittency of renewable electricity and finding carbon-neutral resources are major causes of this materials transition.
Electric vehicle (EV) batteries are an interesting example of this materials transition. In a world where hundreds of millions of cars run on gasoline, we must produce and process a significant amount of petroleum. Entire industries are dedicated to this effort. However, in a future world where most cars are powered electrically, we must build new industries to produce and process the metals used in EV batteries.
While studying this subject, I have noticed that those active in this materials transition often refer to the three variables of abundance, concentration, and cost to describe their work (Fig. 1). These variables are interrelated. Engineers always have to make tradeoffs between interrelated variables to accomplish their goals. New companies have arisen within the past few years to make novel and innovative tradeoffs between these variables. In this post, I want to discuss how Lilac Solutions, KoBold Metals, and Electric Hydrogen each address the tradeoffs between these variables for their respective businesses.
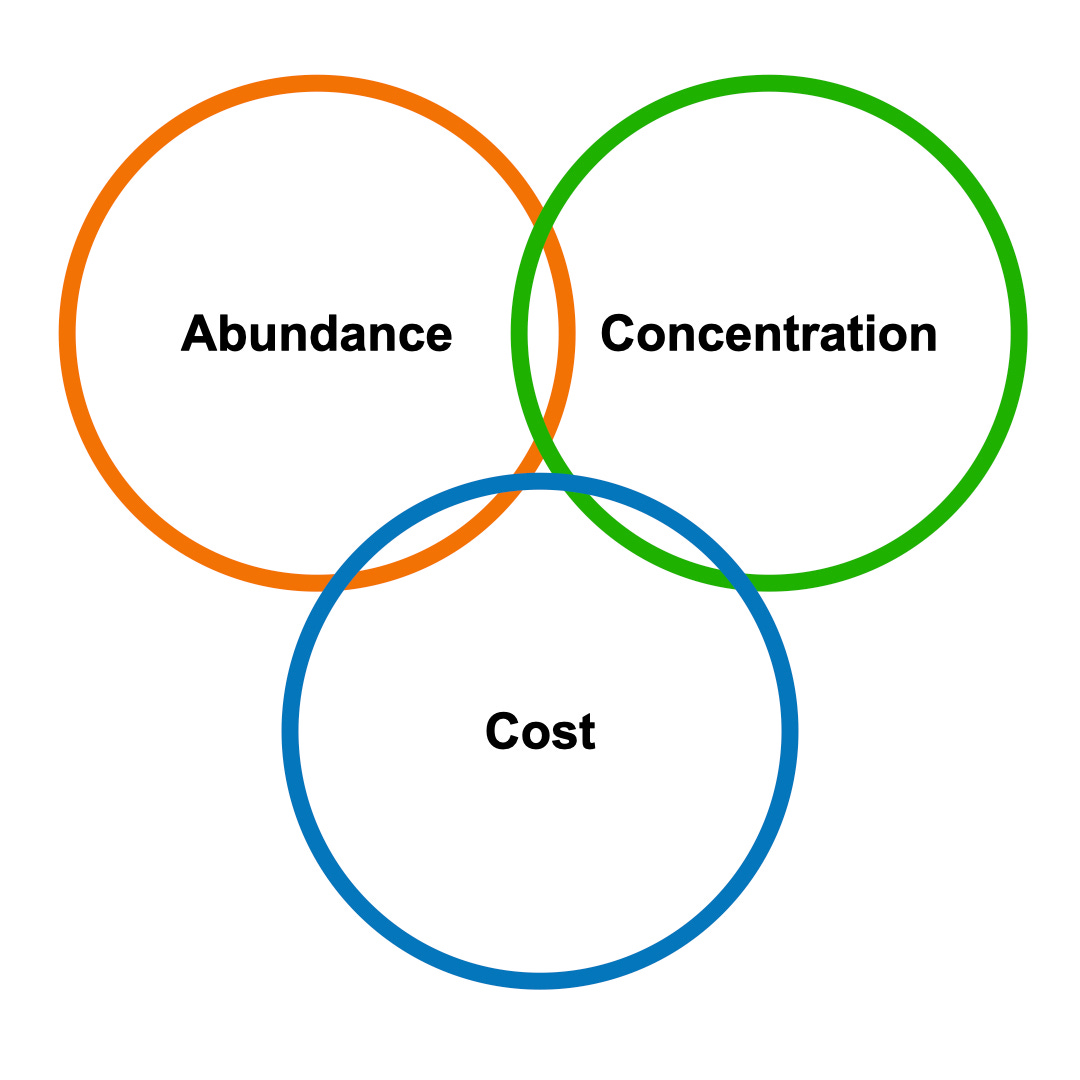
Lilac Solutions
Lithium (the third element on the periodic table) forms the lightest and smallest metal ion, which makes it ideal as the charge carrier in EV batteries. For this reason, lithium-ion batteries have the highest energy density amongst common battery formulations. A typical electric sedan or light truck may have 50 kg (110 lbs) of lithium in the battery pack. The current lithium supply is inadequate for producing tens of millions of new EVs in the coming decades.
Lithium is either extracted from hard rock or from underground brine solutions. The traditional method of extracting lithium from brine involves pumping lithium-rich brine solution into large overground pools (Fig. 2). The brine solution is then further concentrated through evaporation (a process that can last multiple years!). Lithium is actually an abundant metal. There is even lithium in ocean water. However, at a concentration of 0.17 mg/L, 300 million liters (120 Olympic swimming pools) of ocean water would be necessary to produce a single EV. Processing that much ocean water is too costly to be economical.
The highest concentrations of lithium brine are found in underground reservoirs beneath arid high-elevation dried lakebeds. This geological formation is most common in the border region between Bolivia, Chile, and Argentina. Lithium mining is economically relevant in this region because the brine solution has become highly concentrated through natural means. At a concentration of up to 1,400 mg/L, 36,000 liters (1/70th of an Olympic swimming pool) of brine solution from this region produces enough lithium for a single EV.
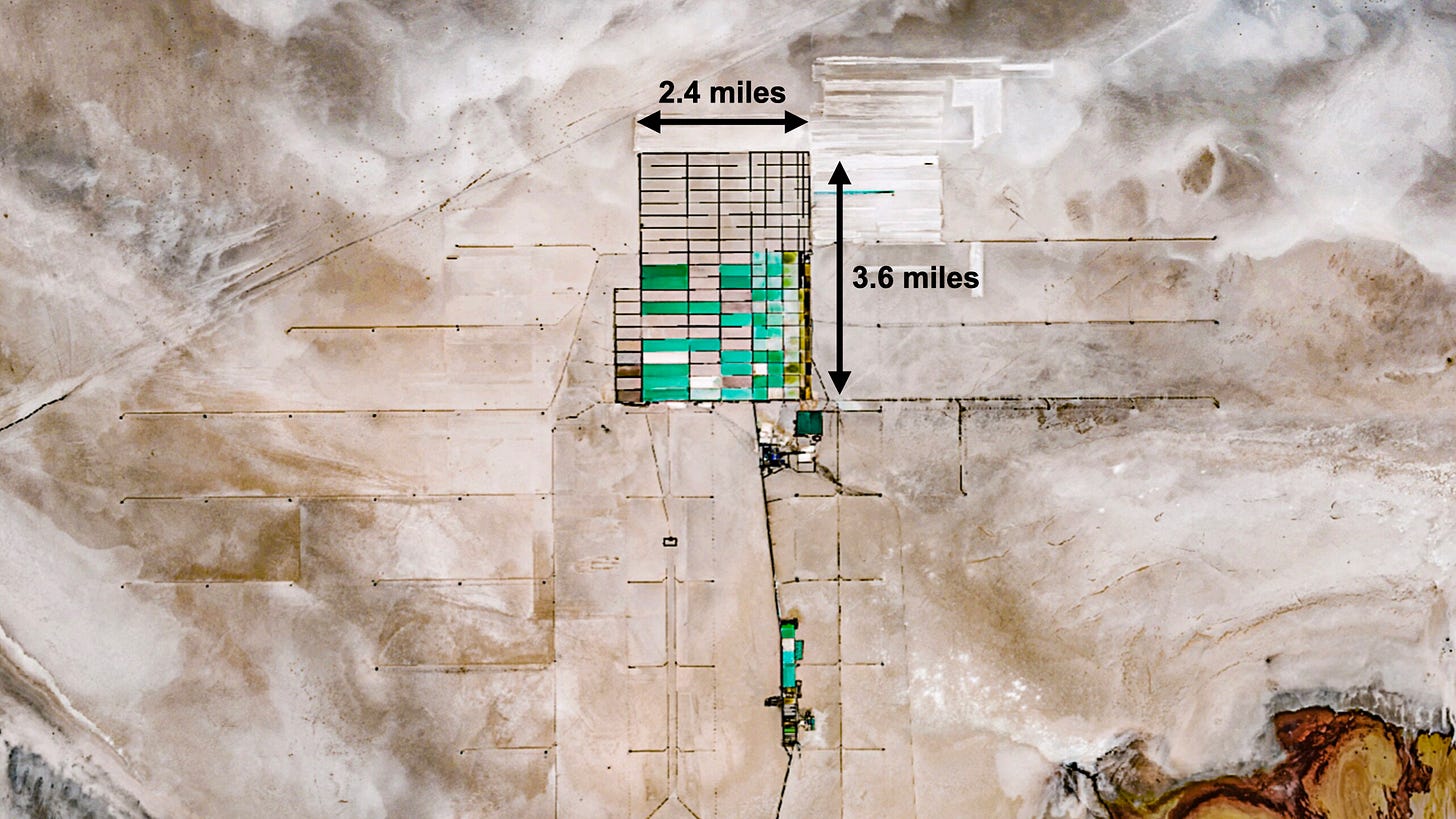
We already see the tradeoff between the variables of abundance, concentration, and cost in this example. Ocean water is much more abundant than the geological features that give rise to lithium-rich brine solutions beneath dried lakebeds. However, sourcing lithium from ocean water is very costly because the concentration of lithium in that solution is so low.
Lilac Solutions addresses this tradeoff in a new way. Dave Snydacker, the founder and CEO of Lilac Solutions, told My Climate Journey (MCJ),
For people who have studied oil and gas, or mineral resources, there's this classic pyramid where at the top you have a small number of sites with a very high-grade resource, and as you go down the base of the pyramid, you get to lower concentrations of lithium, but a much larger resource base with more sites that can be developed. And so, outside of the existing production, which was three or four brine projects, there were dozens of sites with large amounts of lithium, but at a lower concentration, where conventional technology would not be applicable. And it's those sites that seemed like the frontier for lithium.
Lilac aims for a more abundant resource, which is brine solutions with a slightly lower concentration of lithium. They overcome the lower concentration with a process that concentrates the lithium faster than traditional methods. They use ceramic beads that absorb lithium from the brine. They subsequently wash these beads in hydrochloric acid to produce lithium chloride. They process this salt into lithium carbonate or lithium hydroxide, which are the raw materials needed for batteries. While the additional processing adds cost, it gives Lilac access to much greater reserves of lithium.
KoBold Metals
KoBold Metals is another company active in the materials transition associated with electrification. They aim to increase the supply of copper, nickel, cobalt, and (hard rock) lithium. The world needs $15 trillion (!!!) of these materials by 2050. These metals are important in conducting electricity and building energy-dense EV batteries.
In his interview with MCJ on the Stanford campus, Kurt House, the co-founder and CEO of KoBold Metals, noted that,
I would take a step back actually and think about what a[n] ore deposit actually is. So you could dig down underneath Stanford's campus right now and you'll find cobalt guaranteed… You'll find cobalt probably around 20 to 50 parts per million… [There’s] something like 10 to the 18th kilograms of cobalt near Earth’s crust…
The problem is it's in this low, very low concentration background concentration, and so to try to extract it from that background concentration would be way too expensive… An economic ore deposit is when you find a chunk of earth that doesn't have 30 or 40 parts per million cobalt, but rather it has 20,000 parts per million cobalt. That's what you want.
Kurt House is referring to the same resource pyramid that Dave Snydacker mentioned. However, KoBold pursues a different strategy compared to Lilac. Whereas Lilac moves down the concentration pyramid to increase abundance, KoBold increases abundance by finding concentrated metal deposits deeper in the Earth’s crust (Fig. 3). They do so partly by modeling the geological features and soil content that led to previous discoveries, and by making more sophisticated decisions about where to drill test wells. This effort, which is enabled by advances in artificial intelligence, allows KoBold to reduce the cost of discovering rich ore deeper in the Earth’s crust. A similar tradeoff between abundance, concentration, and cost holds here as well.
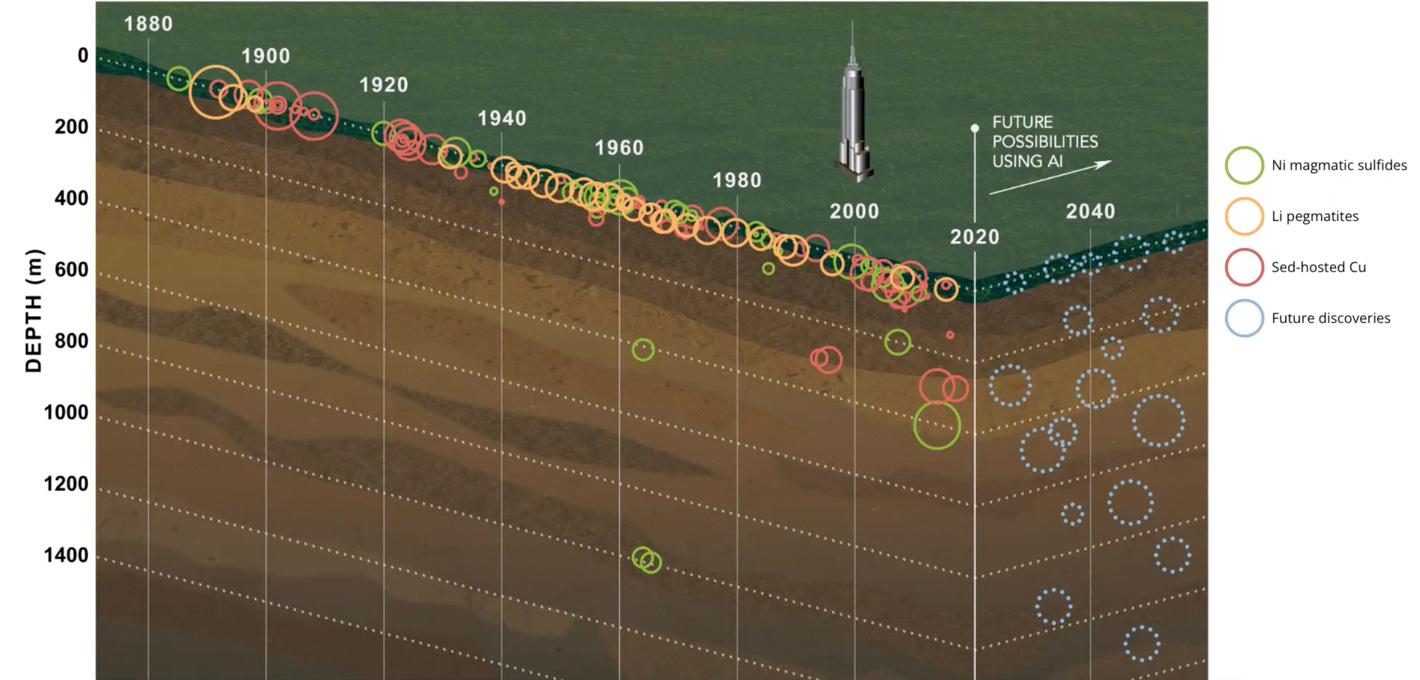
Electric Hydrogen
Electric Hydrogen (EH2) is also involved in the energy and materials transition, albeit not in electrification. EH2 will start by addressing an existing market, which is in supplying industrial-scale hydrogen for the production of fertilizer and petrochemicals. However, it expects that in the future there will be a much larger market for hydrogen as a way to store chemical energy, and it intends to address that market as well.
Industrial-scale hydrogen is currently primarily produced through methane steam reforming (MSR). In this reaction, methane (CH4) endothermically reacts with steam (H2O) in the presence of a catalyst to produce hydrogen (H2) and carbon dioxide (CO2). The same variables of abundance, concentration, and cost are relevant here. Methane is abundant (in the form of natural gas) and it is a concentrated source of hydrogen (25% by mass). In addition, MSR is a relatively inexpensive process.
EH2 uses water (H2O) as its source of hydrogen atoms, which is abundant. Water is also a relatively concentrated source of hydrogen (11% by mass). EH2 produces hydrogen using the water electrolysis reaction, where water is split into hydrogen (H2) and oxygen (O2) with the application of electrical energy to an electrocatalytic stack.
The main drawback of MSR is the production of CO2, which is a global warming agent. The wider adoption of MSR over electrocatalytic water splitting relates to the cost required to access that hydrogen. Historically, most of the electricity in the US came from power plants that burned coal or natural gas. The hydrogen produced from electrocatalytic water splitting using this electricity was far too expensive compared to the hydrogen produced from MSR. However, EH2 is benefitting from one major trend and two innovations:
Solar and wind power are not only carbon-free, but also they are now less expensive than power produced with fossil fuels.
EH2 has increased the efficiency of its electrocatalytic stacks with device physics innovations.
EH2 is building much larger electrolysis plants (100 MW instead of 25 MW) to reduce costs using economies of scale.
Combining these three elements, EH2 is aiming to lower the cost of its electrolytic hydrogen to be on par with hydrogen produced using MSR. In his interview with MCJ, Raffi Garabedian, the co-founder and CEO of EH2 said that, “we're running as fast and as hard as we can towards the capability to produce at fossil-parity. That means under $1.50 a kilogram of hydrogen produced. We think that that's possible in the next few years.” In this case, the main difference between new and traditional methods is in the cost and not in the abundance or the concentration. However, we can still analyze this innovation using the same three variables, where producing hydrogen at “fossil-parity” cost is the main challenge for EH2.
Conclusion
The world is poised to experience a materials transition in parallel with the energy transition of the coming decades. In this materials transition, there is a tradeoff between the abundance and concentration of materials and the cost to produce or process them. Lilac Solutions, Kobold Metals, and Electric Hydrogen have each addressed these tradeoffs in a unique way (Fig. 4).
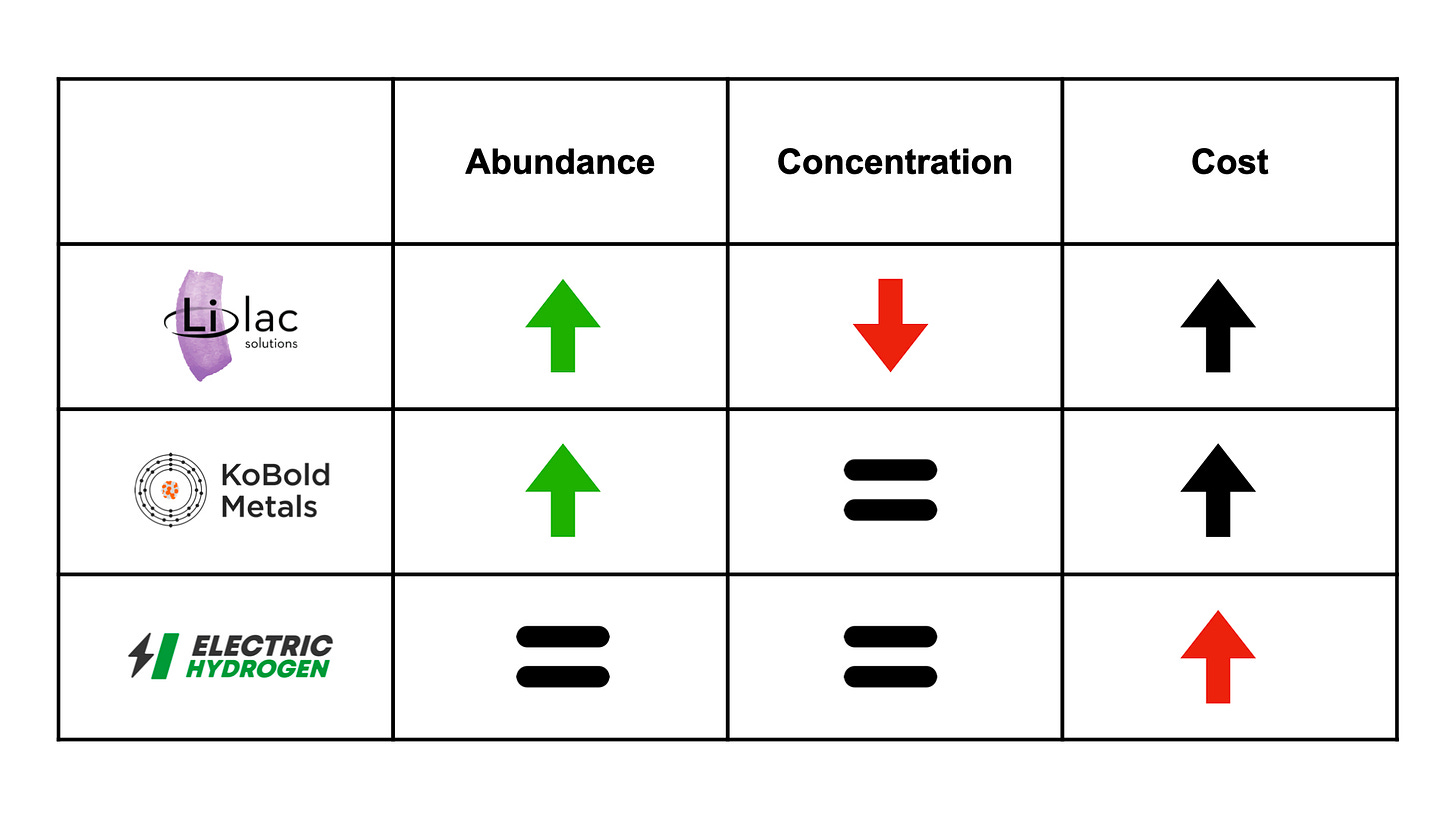
Lilac’s technology allows them to extract lithium from less concentrated but more abundant brine resources. KoBold’s technology allows them to find concentrated mineral ores deeper in the Earth’s crust, where they are more abundant. For both companies, production costs from these new resources will likely be higher than traditional resources. However, these metals are in such high demand that the new operations will still be economical. We see the same principle in the extraction of other resources. For example, on-shore petroleum from the Middle East costs $31/barrel to produce, whereas hydraulic fracturing petroleum from North America costs $44/barrel to produce. In August 2023, when each barrel of petroleum sells for $82, both operations are profitable.
EH2 is extracting hydrogen from water instead of methane. There is no significant difference in the abundance or concentration of methane or water. Historically, producing hydrogen through water-splitting was much costlier than MSR. However, EH2 is taking advantage of new industry and technology trends to reduce this cost difference to parity. Cost parity is what allows them to substitute electrocatalytic hydrogen for MSR hydrogen.